Communications
Overview
![]() Deployable Antenna2 |
There are a multitude of issues to be addressed when designing a communications link to Epsilon Eridani. Because electromagnetic radiation decreases with a factor of 1/r2, the overwhelming distance of 10.5 light years is the major area of concern when constructing our link budget. To overcome this problem, a combination of high powered transmission along with an extremely high gain antenna will need to be employed. Each aspect of the link budget will be analyzed to find the best engineering solution to the overall problem: |
Below is the standard Link Budget Equation:
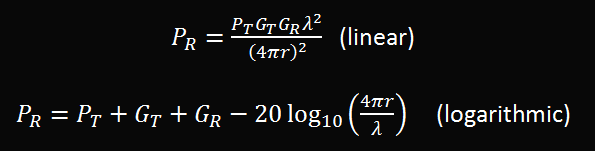
Each of these components of the link budget will be optimized below:
Frequency of Transmission
Directivity Gain
As seen in the link budget, the power received is a function of λ. As wavelength increases (decreasing frequency), the power received actually decreases by squared factor. However, since there are have 2 Gain terms that increase with frequency squared, a higher frequency will ultimately result in an overall higher received power. Thus higher frequencies are preferred with point-to-point satellite communications links. Below is a table of frequency bands commonly used for space communication:
S-band | 1.55-5.2 GHz |
X-band | 5.2-10.9 GHz |
Ku-band | 12-18 GHz |
Ka-band | 20-40 GHz |
Even though higher frequencies are theoretically better, there are other limitations to take into account. Because of the sheer distance of the link,a frequency must be chosen with the least amount of noise. The following graph shows the quietest portion of the electromagnetic spectrum1:
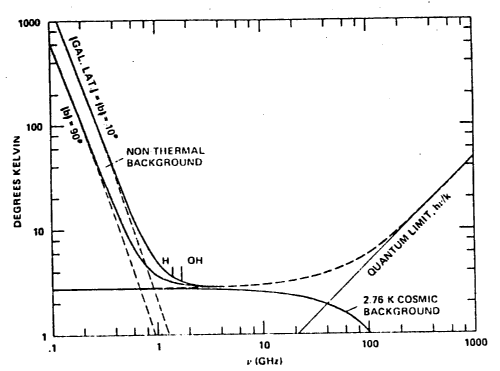
There are three fundamental sources of noise for sensitive radio receivers. There is galactic noise which rises steeply below 1GHz, there is thermal noise that is roughly 3K, and there is also quantum noise at higher frequencies. From this graph the communications link would have lowest amount of noise in the range of 1-60 GHz.
In addition because the receive antenna is inside the earth’s atmosphere, there will be additional losses from the air the signal will need to travel through. The following graph shows the effect of atmospheric absorption at different frequencies1:
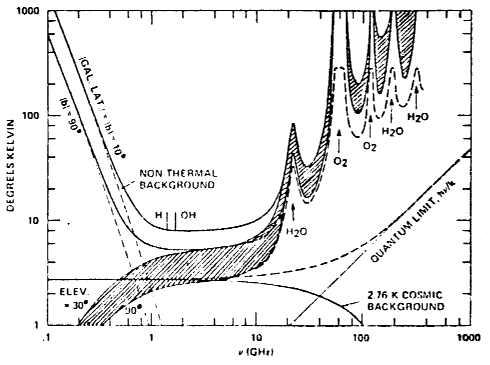
This limits the best frequency choice to within 1-10 GHz. Thus the high end of this range-10GHz will be chosen for the communications link. At carrier frequency of 10 GHz, the free space path loss for traversing 10.5 light years (9.93x1016 meters) is a substantial:

This overwhelming path loss is overcome with the following factors:
Transmit Gain
Deployable Antenna
As discussed in the Electronics Section, as the Bound Vehicle gets further away, latency will be a huge problem. Thus it will need to travel autonomously and all course correcting and adjustments will be made on board. This also means that only a downlink is required to send the images upon arrival at Epsilon Eridani. When the Bound Vehicle arrives it will automatically deploy a large parabolic folding dish to act as the transmit antenna. There are already designs in use with diameters that approach 30m such as the Harris Advanced Hoop-Truss Reflector2:
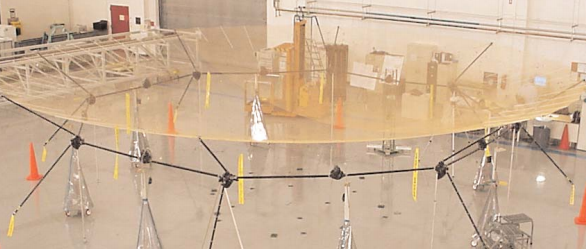
With this circular parabolic dish at a carrier frequency of 10 GHz, and assuming a modest 50% efficiency, the Transmit Gain would be:
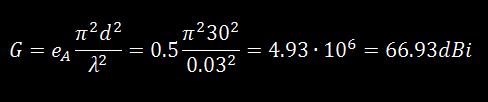
Receive Gain
Radio Interferometery
The earth station is much less limited in terms of power and size so this is where most of the path loss needs to be made up . However there are a number of factors to consider.
First there is the cutting edge for antenna aperture size. The state of the art right now is the Five hundred meter Aperture Spherical Telescope (FAST) antenna to be completed in China in 20133. As its name suggests its antenna size will be 500m in diameter but will actually be steerable unlike the current 305m antenna in Arecibo, Puerto Rica. However, FAST's operating frequencies are 0.3 to 5.1 GHz which is slightly lower than desired.
In addition, constructing very large antennas is a problem because of surface roughness. With relatively high frequencies such as 10 GHz, the wavelength is about 3cm. This is a problem especially with very large antenna because, as there are slight mechanical deformations in the dish, they are on the same order of magnitude as the wavelength and thus will cause scattering of the waves. This is likely why the operating frequencies of FAST are limited to 5.1 GHz. However, the problem can be somewhat compensated for with a concept called radio interferometry4. By arraying smaller antenna together and using a central processing facility to simulate a much bigger antenna. The state of the art with radio interferometry is the Square Kilometre Array project (SKA). Set to be fully constructed by 2024, the SKA will combine the signals of thousands of small antenna to achieve an effective diameter of more than 1000m4:
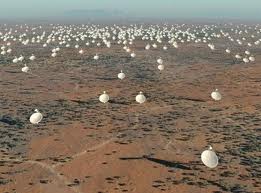
Finally, because radio waves do not pass through solid objects very well, the satellite must maintain a direct line of sight with the earth station in order to sustain communications. This is problem because the Earth rotates and the earth station may be on the other side of the planet. Luckily, NASA has already solved this kind of problem with their Deep Space Network5. THE DSN has 3 stations on Earth spaced 120 degrees apart in longitude. Each station has large 34m and now 70m diameter steerable antennas to provide constant service for deep space probes.
Despite these problems, because of the travel time needed for the the Bound Vehicle to reach Epsilon Eridani, we can extrapolate for much better material science technology before even constructing the receive antennas. It is definitely difficult to extrapolate a millennia into the future, because there could be some breakthrough quantum technologies that could change everything. But with a very conservative estimate based on the SKA, the receive antenna will have an effective diameter of 1000m thus the receive gain assuming 50% effciency will be:

Transmit Power
Using Reactor
Because of the sheer distance, launching relay satellites were initially considered to help achieve the communications link. From the Propulsion section it was found that a mini fission reactor capable of providing 250KW of power was needed to drive the ion drive. Upon arrival at Epsilon Eridani, the ion drive no longer needs to be powered. Thus much of much of the power that was powering the ion drive can be directly shifted to transmit power. However some power will still be needed to heat and power the data-gathering electronics. Thus a conservative estimate for transmit power is 50KW or 46.99dBW. This power is more than enough to avoid the the added complexity and cost of relay satellites.
The estimate for the overall receive power of the signal received is:
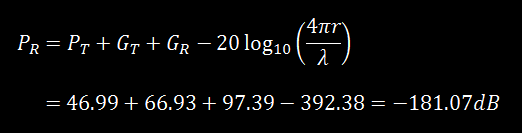
Even though this is an extremely small amount of power as long there is a low enough received noise communications is possible.
Received Noise
Low Bandwidth
The equation for power of noise received is:

K is Plank's constant and T is conservatively estimated as 20K on because it is just the background radiation of the universe plus the system noise of a futuristic receiver. Based on this equation limiting, the bandwidth will limit the amount of noise received which is critical to achieving an acceptable SNR. Using a very narrow bandwidth of 200Hz and accounting for a possible 6dB extra of losses our received noise is:
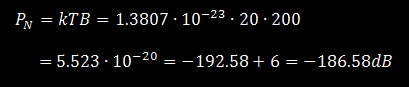
Bit Error Rate & Data Rate
BPSK
Based on the power received and the noise received the SNR of the communications links will be:

Using uncoded BPSK the BER would be:
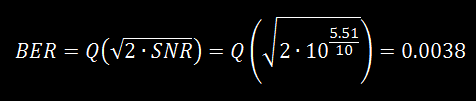
A BER of 0.0038 results in losing 1 in every 263 bits which is still substantial and due to latency it would be impossible to request for a retransmission of lost bits. Thus a forward error correcting code is used. The most powerful of these is the turbo code with a BER that is extremely low for any SNR over 1dB. A rate 1/2 turbo code could be used to result in a virtually error-free communications channel. As far as data rate. From the Shannon-Hartley theorem, the ideal channel capacity for the 5.51dB SNR is:

However BSPK is 9.6dB away from channel capacity6. Reusing the formula gives:

Conclusions
Conservative Estimate
Based on current technology the Bound Vehicle can be launched with a 30 meter deployable dish antenna. By the time it reaches it Epsilon Eridani and with conservative estimates for receive antenna technology, we will achieve a data rate at the minimum of 94 bits/second. With this data rate a high resolution picture of 10 Mb will ultimately be downloaded in just under 30 hours.