Power System
A number of power systems are available for space vehicles, with varying benefits and drawbacks. A summary of available power sources, according to power output and duration, is shown below.
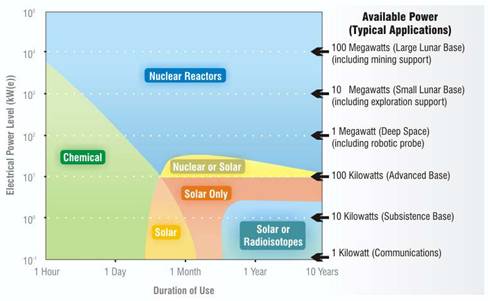
The power system design has considered the following power collection and generation techniques:
1. Radioisotope Thermoelectric Generators (ref 2):
RTGs tend to output 3 W to 300 W of power using Plutonium-238, which is currently in short supply, and has a half-life of approximately 88 years. Other fuel sources are available with shorter half-lives, such as Strontium-90 (28.8 years), Polonium-210 (138 days), and Curium-242 (163 days). Radioactive decay in a RTG is shown below. The Cassini spacecraft carried 3 RTGs on its mission to Saturn, capable of providing 870 Watts of power.
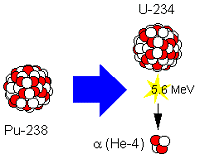
2. Solar Arrays (ref 4):
Solar arrays are limited to operation near a star, since the intensity of light varies inversely to the square of the distance from the star. The largest solar arrays to have flown in space output approximately 50 kW. A typical power-to-weight ratio is 80 - 100 W/kg, with most commercial satellites being restricted to a 200 kg mass allocation (i.e., 20 kW). Current solar arrays are only about 30% efficient at the beginning of life, although development of advanced solar cells indicates that a 50% efficiency is attainable in the near future.
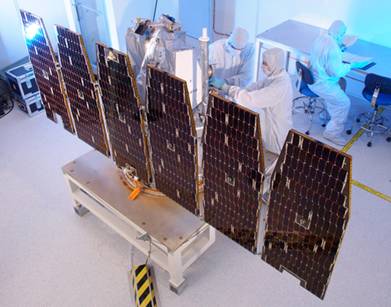
A space solar array developed by the Orbital Sciences Corporation (ref 5):
3. Batteries (ref 6):
Rely on constant recharges to maintain power output. A typical Lithium-Ion space battery has a 50 ampere-hour capacity at 175 watt-hours per kilogram. The lifespan of such a battery is approximately 18 years.
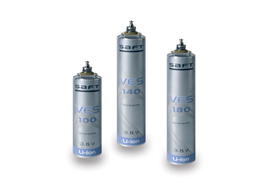
Lithium-Ion space batteries, model VES by the Saft Corporation (ref 6)
4. Nuclear Fission Reactor (ref 7):
Nuclear fission has the potential to produce over 100 kWe. The SNAP-10A reactor was launched in 1965 and produced 45 kWt and 650 watts electrical using a thermoelectric converter. By 1990, the Topaz project aimed for 40 kWe, although budget restrictions forced cancellation of the project. A nuclear fission reaction is shown below.
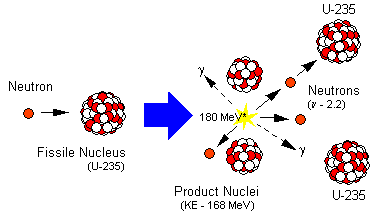
Decay of Uranium-235 in a nuclear fission reactor (ref 3)
5. Heatpipe Power System (ref 7):
Heatpipe power involves transferring energy from a fission reactor core directly to a Stirling cycle or Brayton cycle converter. Development of these systems has been ongoing since 1994 at the Los Alamos National Laboratory. The SAFE-400 space fission reactor uses uranium nitride to produce 400 kWt, or about 100 kWe, with a 512 kg core mass and 72 kg mass for each heat exchanger. Although the SAFE-400 is not completely developed yet, similar models (e.g., SAFE-100A and SAFE-30) are in development to prove the concept.
Requirements Discussion
The principle requirements of a power system for the Epsilon Eridani mission are long life, ability to operate away from solar energy, and high power output upon arrival at the exoplanet. The overall mission timeframe will span many centuries based on modern propulsion technology. Since the mission will spend a significant amount of time in interstellar space, away from the Sun and Epsilon Eridani, it cannot depend entirely on solar power. Due to the large distance between Earth and the exoplanet, communications will require a very large amount of power (10s of kilowatts) upon arrival.
Considering the requirements, some of the power alternatives are less feasible than others. Battery power does not make sense given the long mission duration, although it could be used for temporary energy storage. Solar power is only an option within the solar system or near Epsilon Eridani; a stowable or inflatable solar array might provide an added boost of power at the destination. RTGs are not practical given the communications system power requirement. Nuclear fission reactors, in the classical sense, also produce too low of an electrical power output to meet the mission requirements, due to thermoelectric conversion inefficiency.
Power System Design Conclusions
The Heatpipe Power System with a nuclear fission reaction is the overall best option for a long-duration mission to Epsilon Eridani. The fuel source, Uranium-235, has a half-life of 700 million years. If a design similar to SAFE-400 is assumed and 4 heat exchangers are used, the reactor will produce 100 kWe with only an 800 kg mass.
A typical nuclear fuel rod can last several years before it must be replaced (8). The power solution for an Epsilon Eridani mission will need to have a sufficient number of fuel rods that can be inserted or ejected automatically as needed during critical mission periods. A small bank of capacitors can be used to power the system to handle the fuel rod transitions; the capacitors will automatically be charged as reactor power output decreases, provide power to the insertion/ejection system, and then remain dormant until the reactor output decreases again. These capacitors will have negligible weight relative to the rest of the spacecraft.
The mission power profile is shown below. Power requirements for the first several years will be high to power the electric propulsion system. Once the proper cruising velocity is reached, power requirements are significantly lower until the propulsion system must again be activated to slow down near the Epsilon Eridani system. Finally, near the end of the mission, the spacecraft will enter orbit around Epsilon Eridani B and require large amounts of power to transmit information back to earth.
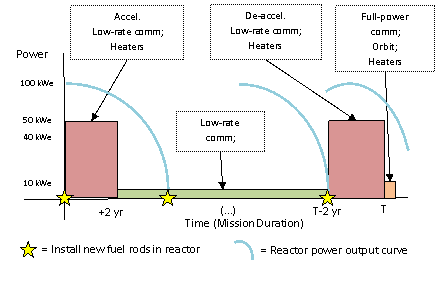
References
(1) Campbell, Michael D., et al. “The Role of Nuclear Power in Space Exploration and the Associated Environmental Issues: An Overview”, EMD Committee Meeting at AAPG Convention, Denver, Colorado, June 7-10, 2009.
(2) Teofilo, Vincent L., “Space Power Systems for the 21st Century”, Lockheed Martin Space Systems Company. Link
(3) Netting, Ruth. “Space Power”. Link
(4) Reed, Kevin, and Harvey Willenberg “Early Commercial Demonstrations of Space Solar Power Using Ultra-Lightweight Arrays”. Link
(5) Boone, Katy (ed). “AIM Solar Array Development”. Link
(6) Saftbatteries.com “Lithium-Ion: Space VES Cell”. Link
(7) “Nuclear Reactors for Space”, The Encyclopedia of Earth, December 6, 2009. Link
(8)“Energy Resources: Nuclear Power”. Link